Hydrostatic drives are in service for more than 60 years, predominantly in heavy construction machinery where the interruption of torque during shifting is not admissible. Because of their ease of use, they became also popular in garden tractors and other small equipment. However, speed range and efficiency are here of lesser importance. The drives consist of a combustion engine driving a pump, transmitting pressurized fluid to a hydraulic motor which converts the energy back into mecha-nical power. Pumps and motors have a variable displacement to extend their range of operation. The ability to store and release energy fast increases the power and efficiency and reduces the required size of the engine, the consumption and emissions.
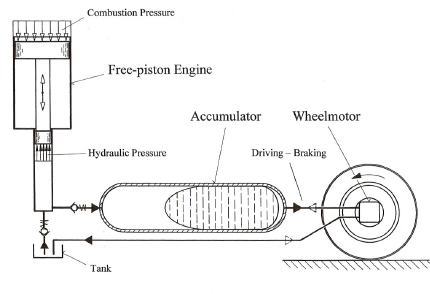
Concept of Hydrostatic Drivetrain
The drives are not suitable for road vehicles since their operating range is by far too small and the energy losses, costs and weight are too high. Only drastically improved technical data and a reduction in costs allow for vehicle concepts fulfilling the requirements for the considerable reduction in fuel consumption and emissions. In addition, measures which improve the safety should be included.
The new advanced hydrostatic drive fulfills these requirements. It consists of a hydraulic Free-Piston Combustion Engine for producing pressurized fluid, an accumulator for storing the energy, and hydraulic Axial-Piston Wheel Motors for driving and breaking the vehicle.
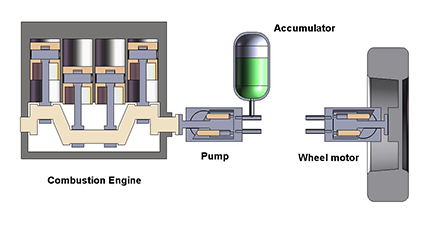
Conventional Hydrostatic Drivetrain
Since the accumulator provides the power peaks for acceleration only a noticeably smaller engine (-2/3) is required to charge the accumulator. The intermittently working engine operates here at optimal conditions (load, speed) for best possible fuel consumption and emissions. Other types of energy sources (electric, mechanic) can also be applied to charge the accumulator.
The development of the Hydraulic Hybrid ‘Ingocar’ is based on previous tests of the hydraulic motor and detailed designs of the new free-piston engine. The drivetrain technology includes a larger number of features described in Patents (6) or Patent applications (2). Their influence in the Ingocar, its drivetrain components, and additional advanced hydro-static drive systems are demonstrated in the following sections.
Hydraulic Free-Piston Combustion Engine
a. General advantages: Small, lightweight, proven materials and manufacturing processes, multi-fuel capability.
b. Less friction: No crankshaft and connection rods, no valve train, no piston side loads, fewer moving parts.
c. Highest combustion efficiency: High Compression Ratio and Pressure-Wave Charging, konstant speed and power, peripheral fuel injection with 50,000 psi, compact, ceramic coated combustion chamber, recuperation of exhaust energ (pressure & heat).
d. Lowest emissions: 33% less CO2, 70% less NOx, low soot (Hydraulic Hybrid 33 gr/100km CO2, no braking dust, 1/3 tire dust).
e. Overall efficiency: 63%, fuel consumption 0.227 lbs./hph (Hydraulic Hybrid 190 MPG).
The Hydraulic Free-Piston Engine with Impulse-Charger is very simple, small, and efficient. The combustion forces are directly transferred into hydraulic forces, without utilizing a crank mechanism. The piston is free of side forces and its lubrication is not required. The mechanics are therefore insensitive towards high pressure, temperatures and veloci-ties. Therefore, high charge pressures and compression ratios can be used, achieving unusually high powers and efficiencies and simulta-neously a significant reduction in size.
The 2-stroke free-piston engine has two opposing pistons in one cylinder. Position and velocity of the pistons are determined through the combustion and hydraulic forces acting on the pistons. The forces are initiated through fast, electro-hydraulic vales, controlling at the end of the stroke, independently from each other, the opening and closing of the in- and outlet ports for the combustion air. This allows the best type of scavenging (uniflow) and a vibration-free operation. The opening and closing of the ports and the compression ratio (up to 35:1) become fast and infinitely adjustable, allowing the use of all common combustible fuels (fluids, gases) under optimal conditions. This control of ports, uniflow scavenging, and direct fuel injection overcomes the disadvan-tages of the principally simpler and more efficient 2-stroke system.
The piston of the impulse-charger is driven by the exhaust gases of the engine and extracts the thermal and kinetic energy form the exhaust gas very efficiently (pulse charge, low thermodynamic losses). The air side of the charger piston pumps compressed air to the combustion chamber, and the hydraulic side pressurized fluid into the accumulator. The distribution in charge air and hydraulic power is infinitely adjustable to maximize the energy extraction form the exhaust gas. The stepwise reduction of the gas pressure reduces the exhaust pressure and radiation of noise.
The p-V Diagram shows the high compression and power of the free-piston engine and the recuperation of exhaust energy in the additional expansion phase of the impulse-charger.
The hydraulically driven fuel injection pump of the new peripheral fuel injection system transmits the hydraulic pressure of the accumulator directly and very efficiently into very high pressure (50,000 psi) of the fuel. The fuel is injected through a larger number of very small slots (20) at the circumference of the combustion chamber. The peripheral arrangement prevents the insufficient local air supply due to the con-centration of fuel beams (5-8) at the nozzle. In addition, an impinge-ment of fuel at the cylinder wall and piston bowl, not required anymore, is largely prevented. The resulting, homogeneous distribution and high atomization of fuel reduces high local temperatures during the com-bustion. Highly turbulent air, noticeably increasing the heat losses and thermal stress for piston and liner is not required anymore, increasing the efficiency. Under these conditions, NOx (>70%) and soot emissions are significantly reduced. In the meantime, nearly non-measurable NOx emissions have been reached at limited operating conditions with com-parable, but less sophisticated systems (KIT, SWRI). The HC and CO emissions are generally relatively low in Diesel engines, and CO2 emissions are reduced proportionally to the fuel consumption (>30%).
Diesel engines of comparable design for large ships (2-stroke, stroke to bore ratio > 3:1, uniflow scavenging, turbo compounded) achieve high conversion efficiencies of up to 55% (251 lb/hp·h). A large ship engine (MAN B&W K98MC) achieves at a low piston velocity of 27 ft/sec (8.3 m/sec), very poor fuel quality, and a mean effective pressure (b mep) of 264 psi (18.2 bars) a ‘piston area power’ of ca. 6.6 hp/in² (755 W/cm²) (Mollenhauer, Handbuch Dieselmotoren, Springer Verlag, 2001, ISBN 3-540-41239-5) The specific power per piston face area (hp/in²) indi-cates the State of the Art of combustion engines, independent of its size and rotational speed. It is also worth noting that the cylinder barrel of the engine is here not water cooled.
The low sensitivity of the free-piston engine towards high pressures, temperatures and velocities (no piston side loads), the noticeably higher quality of the fuel and combustion, and the high efficiency of the impulse-charger allow for a significant increase of the above stated power of 6.6 hp/in². An increase of the piston velocity to 50 ft/sec and a b mep to 650 (+) psi, and higher charge pressure and increased com-pression ratio allow for a ‘piston area power’ of 30 hp/in². These data are used for the layout of the engine for the helicopter and the tracked vehicle. It is expected that the favorable dimensions of the large Diesel engines (low cylinder wall heat losses) are more than compensated through the high combustion efficiency and reduced mechanical friction of the free-piston engine. Current engines for cars in production reach performances of 2.96 hp/cu.in (133 kW/L) (Mercedes AMG), a b mep of 464 psi (32 bar) (BMW, Borg Warner) and velocities of 59 ft/sec (18 m/sec.). Latest engines for rally cars reach 5.56 hp/cu.in (250 kW/L) (VW). It is expected, that the higher rpm. of the current engines will be compensated through the noticeably higher pressures and increased efficiencies of the free-piston engine.
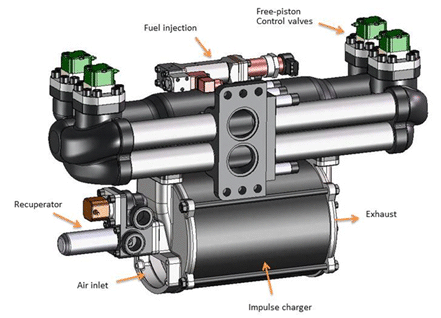
Summary: The expectations of achieving the high thermal efficiency of 65% are based furthermore on the excellent dynamic behavior of the opposing free-pistons (nearly constant volume combustion), smaller surface area (-35%) of the combustion chamber, and highly efficient recuperation of exhaust energy through the impulse-charger. When considering the very low friction losses of the free-piston engine and the additional hydraulic losses (+ ca.5%) for controlling the piston and driving the fuel injection pump, a reduction in specific fuel consumption to 230 lb/hp·h (-30%) (140 gr/kW·h) is expected.
In comparison with Turboshaft engines, the Free-Piston Engine reduces the fuel consumption by 45% and the space required by 55%. The use of Titanium components lowers the weight by 35%. (See table below)
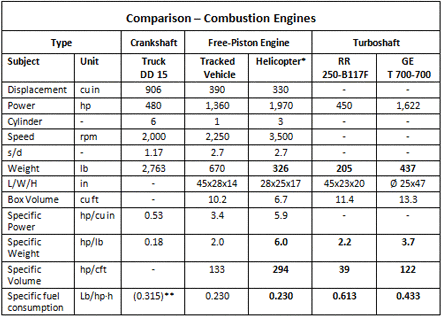
Hydraulic Motor
Hydraulic motors for vehicles have to be very efficient, small, light, and their displacement has to be adjustable. These conditions require high operating pressures, obtained reliably only with piston units. Axial-piston motor types provide, in comparison with radial-piston units, a wider adjustment range, higher rotational speed, and lower weight. Of those, bent-axis type axial-piston motors have a wider range of adjust-ment and speed and are more efficient (lower mechanical losses), but are noticeably larger in size, heavier, more expensive and have a shorter life expectancy than swashplate-type motors.
The new motor is a swashplate type unit. Several patented and new features result in a drastically reduced size and weight (-60%), an increase in speed and a widening of the adjustment range of the displacement, and a significant reduction of losses, especially in the important areas of low powers. The design concept is scalable over a very wide area, from 15 hp to 15,000 hp. In comparison to electric motors for automotive drivetrains, the new motors have a 20 times higher specific power (kW/kg) and a larger factor regarding the specific volume (kW/Liter). In addition, the control for the hydraulic motors is noticeably simpler.
The displacement is infinitely adjusted by tilting the swashplate from 0° to 33° (+57%), comparable with a 40.5° tilt angle for bent-axis type units. However, bent-axis units with large tilt angles have no or only a limited adjustment range and no zero position, as needed for advanced drivetrain concepts. New fluid flow patterns and bearing systems of the new unit widen the speed capacity by ca. 80%. Conventional Hydraulic Hybrids transmit, because of their narrow adjustment and speed range and higher losses, the power at higher speeds through an additional conventional geared transmission and drive shafts.
Based on the new motor geometry, the critical mechanical loads have been lowered noticeably and the mechanical friction losses reduced through double-sided Diamond-Like-Coating (DLC) by more than 50%. The compression losses, significantly increasing with low swashplate tilt angles, are reduced to a minimum due to the off-center swashplate adjustment. Prototype tests have confirmed the efficacy and reliability of the design.
The hydraulic losses are reduced noticeably through several patented and new mechanisms. The small size and shorter sealing areas reduce the external leakage by more than 30%. A significant reduction of inter-nal leakage, generally the largest portion of losses, is reached through patented and new features, i.e. controlling the step-wise adjustment of pressure between the high-, medium-, and low pressure ports at the valve plate. The mechanism also reduces noise and vibration. Tests, experiences, and calculations indicate that the improvements, covering all critical sections, nearly eliminate the advantages of current bent-axis motors and exceed them in the important areas.
Based on the improvements, the operating range - a product of usable ranges for speed, pressure and swashplate adjustment - is increased by a factor of 5.6, and the specific weight (hp/lb) by a factor of 6. The Diagram shows the technical data for the operating ranges of the best current motors in comparison to those of the new motor.
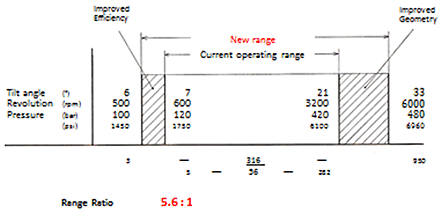
Operating ranges - New and current Hydraulic Motors
The complete front wheel motor has 163 hp (120 kW) and weighs 16.5 lbs with planetary gear stage. The conditions allow for a drive concept where the motor (w/o shiftable gear box) is placed in the wheel hub - small, light, and very efficient.
U.S. Patents, International Patents, Patents pending.
Comparison with electric drives
In comparison with the Ingocar (1.174 lbs), Electro- or Electro-Hybrids weigh 2.5 times as much or more. The energy consumption (Well to wheel) is significantly higher because of the higher weight, significant larger size of the drivetrain, small amount of recuperated braking ener-gy (20%), and the losses from producing and transmitting the electric power. The efficiency of the components (electric/hydraulic) deviate only slightly from each other, however, their number is higher in electric drives. The CO2 emissions are therefore, also when considering future sources of power, higher than for a 190 mpg car.
For practical reasons, electric drives can’t absorb (recuperate) the high amounts of braking energy since current electric motors for passenger cars are 20 times heavier than the new hydraulic motor, and the batte-ries are not capable of absorbing the high power peaks during hard braking. Comparable electric wheel motors would increase the weight of the wheels by about 660 lbs. and capacitors for absorbing the power peaks would increase the already high complexity, costs, and weight of the system.) Therefore, Electric or Electric-Hybrid cars still need a conventional brake system, increasing the space requirements, weight, and costs.
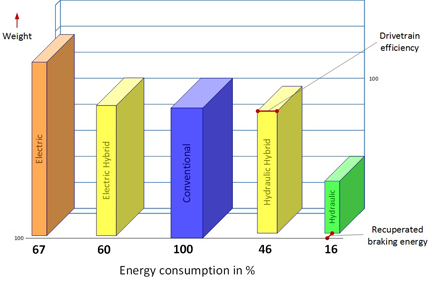
Energy consumption of various car concepts.
The graph shows the influence of weight, drivetrain efficiency and energy recuperation of various drivetrain concepts, approximating the Tesla S, Toyota Prius, a conventional Car, a hypothetical Hydraulic Hybrid with mechanical drivetrain, and the Hydraulic Hybrid - Ingocar.
The comparison becomes more unfavorable when including the increasing dependency on foreign sources of raw material (Metals - Copper, Lithium, and others) and the high expenses for producing, replacing and recycling the batteries. The insufficient travel distan-ces, power supply at low temperatures and incomplete infrastructure are known drawbacks.
Result: The impact on the environment and the costs for buying, operating and maintaining an Electric- or Electric-Hybrid car are noticeably higher – even in long terms.
Additional Applications of Advanced Hydrostatic Drives
It is the goal to significantly improve the function, environmental impact and costs of transportation. The following projects show the conceptual benefits the new technology has in established drive system concepts. The following projects are selected from a wide range of applications:
- High-Speed Helicopter
- Hydrostatic Wind Turbine
- All-wheel-drive Tracked Vehicle
- Locomotive with Energy Storage
- Hydrostatic Marine Propulsion
- Hydro Bike
The goal of this presentation is to obtain financial support for developing the drive system technology and to develop, produce and sell products.